Numerous methods have been used to observe the small spines that extend from neuronal dendrities and make synaptic connections with passing axons. These methods include:
- Golgi impregnation and light microscopy
- Transmission electron microscopy
- Freeze-fracture electron microscopy
- Serial electron microscopy and three-dimensional reconstructions
- Filled cells
- Lipophilic fluorescent dyes
- Intracellular production of fluorescent proteins
- Confocal and multiphoton microscopy
Golgi Impregnation and Light Microscopy
|
Camillo Golgi (1843-1924) |
![]() |
![]() |
Santiago Ramon y Cajal (1852-1934) |
![]() |
Although Santiago Ramon y Cajal made the first observations of dendritic spines (1891) in brain tissue stained with methylene blue according to Ehrlich, his favorite method was the silver impregnation method based on Golgi's original technique. In the hands of innumerable neurohistologists, this method has been admirably fruitful since its introduction by Camillo Golgi (1873), and it continues to be widely used. Golgi and Ramon y Cajal were jointly awarded the Nobel prize for medicine in 1906, for their contributions to understanding the cellular structure of the brain using this method.
The classical Golgi impregnation method is very elegant in its simplicity:
- Immerse a block (approx. 10x5 mm) of formol-fixed (or paraformaldehyde- glutaraldehyde-pefused) brain tissue into a 2% aqueous solution of potassium dichromate for 2 days.
- Dry the block shortly with filter paper.
- Immerse the block into a 2% aqueous solution of silver nitrate for another 2 days.
- Cut sections approximately 20-100 µm thick.
- Dehydrate quickly in ethanol, clear and mount (e.g. into Depex).
Although Santiago Ramon y Cajal made the first observations of dendritic spines (1891) in brain tissue stained with methylene blue according to Ehrlich, his favorite method was the silver impregnation method based on Golgi's original technique. In the hands of innumerable neurohistologists, this method has been admirably fruitful since its introduction by Camillo Golgi (1873), and it continues to be widely used. Golgi and Ramon y Cajal were jointly awarded the Nobel prize for medicine in 1906, for their contributions to understanding the cellular structure of the brain using this method.
The classical Golgi impregnation method is very elegant in its simplicity:
- Immerse a block (approx. 10x5 mm) of formol-fixed (or paraformaldehyde- glutaraldehyde-pefused) brain tissue into a 2% aqueous solution of potassium dichromate for 2 days.
- Dry the block shortly with filter paper.
- Immerse the block into a 2% aqueous solution of silver nitrate for another 2 days.
- Cut sections approximately 20-100 µm thick.
- Dehydrate quickly in ethanol, clear and mount (e.g. into Depex).
Although Santiago Ramon y Cajal made the first observations of dendritic spines (1891) in brain tissue stained with methylene blue according to Ehrlich, his favorite method was the silver impregnation method based on Golgi's original technique. In the hands of innumerable neurohistologists, this method has been admirably fruitful since its introduction by Camillo Golgi (1873), and it continues to be widely used. Golgi and Ramon y Cajal were jointly awarded the Nobel prize for medicine in 1906, for their contributions to understanding the cellular structure of the brain using this method.
The classical Golgi impregnation method is very elegant in its simplicity:
- Immerse a block (approx. 10x5 mm) of formol-fixed (or paraformaldehyde- glutaraldehyde-pefused) brain tissue into a 2% aqueous solution of potassium dichromate for 2 days.
- Dry the block shortly with filter paper.
- Immerse the block into a 2% aqueous solution of silver nitrate for another 2 days.
- Cut sections approximately 20-100 µm thick.
- Dehydrate quickly in ethanol, clear and mount (e.g. into Depex).
The principle of the method is based on the crystallization of silver chromate: the microcrystalline precipitate either grows directly from the surface of the tissue block into transected neuronal processes or spreads from nucleation centers inside the block into nerve cell processes like in preformed channels -- until the neuron has been completely filled. The frequency of nucleation centers is responsible for the unique selectivity of this method, staining only 1-10% of neurons. Without this selectivity, no individual neurons would be seen but only a non-informative overcrowded jungle of cells and processes.
Here is a the Golgi impregnation method in action, and here is a time lapse. More information about the dynamics of Golgi impregnation and its methodical expansions can be found in Harris et al. (1980), Spacek (1989) and Spacek (1992).
References:
- Golgi C (1873) Sulla struttura della sostanza grigia dell cervello. Gazz. Med. Lombarda 33:244-246.
- Ramon y Cajal S (1995) Histology of the Nervous System of Man and Vertebrates. Oxford University Press: New York, English translation by N Swanson and LM Swanson.
- Harris KM, Cruce WRL, Greenough WT, Teyler TJ (1980) A Golgi impregnation technique for thin brain slices maintained in vitro. J Neurosci Methods 2:363-371. (PDF)
- Spacek J (1989) Dynamics of Golgi method: A time-lapse study of the early stages of impregnation in single sections. J Neurocytol 18:27-38.
- Spacek J (1992) Dynamics of the Golgi impregnation in neurons. Microscopy Res Tech 23:264-274.
Transmission Electron Microscopy
Until the era of electron microscopy, dendritic spines attracted little attention. They were even suspected of being an impregnation artefact. E.G. Gray (1959) showed them as real structures on which most synapses are located.
Rather than using light waves, the electron microscope passes electrons through a thin section of tissue. Photographs are made of the variations in electron density within the section. When the tissue has been properly stained, these patterns reveal the fine details of subcellular structures, down to a few nanometers.
The higher magnification of electron microscopy allowed detailed analysis of dendritic spines. In particular, it became possible to address questions of synaptic plasticity by using electron microscopy to evaluate the dimensions of dendritic spines and their synaptic contacts.
For a detailed look at some electron microscopic studies of dendrites see the various components of the Anatomy Tutorials section of this website including the Atlas of Ultrastructural Neurocytology.
References:
- Gray EG (1959) Electron microscopy of synaptic contacts on dendritic spines of the cerebral cortex. Nature 183:1592-1593.
- Gray EG (1959) Axo-somatic and axo-dendritic synapses of the cerebral cortex: an electron microscope study. J Anat 93:420-433.
- Peters A, Palay SL, Webster H (1991) The Fine Structure of the Nervous System. Third Edition. Oxford University Press: New York.
Freeze Fracture Electron Microscopy
Although electron microscopy resolved the issue of whether dendritic spines were real, the method introduced its own complications. Preparation of a soft tissue specimen for transmission electron microscopy involves a difficult process. The tissue must be fixed, stained with heavy metals, dehydrated, and embedded in a compound such as epoxy that can be hardened for thin sectioning. The tissue must be cut into ultrathin sections for high resolution transmission electron microscopy. This sectioning precludes the direct observation of extensive cell surfaces or 3D structure.
Some of these problems were mitigated by the development (in the 1960's) of freezing methods of tissue preparation followed by electron microscopy. In the freeze-fracture method, the frozen tissue is fractured to produce a surface for investigation. The surface is plated with platinum and imaged in an electron microscope. The result is an image of the fractured surface, often revealing parts of dendritic spines.
Unfortunately, as with ultrathin section electron microscopy, the entire 3D structure of a dendritic spine along with its intracellular components cannot be readily determined from the imaged surface.
References:
- Sandri C, Van Buren JM, Akert K (1977) Membrane Morphology of the Vertebrate Nervous System. A Study with Freeze-etch Technique. Progress in Brain Research, Volume 46, Elsevier Scientific: Amsterdam.
- Harris KM, Landis DMD (1986) Membrane structure at synaptic junctions in area CA1 of the rat hippocampus. Neuroscience 19:857-872. (PDF)
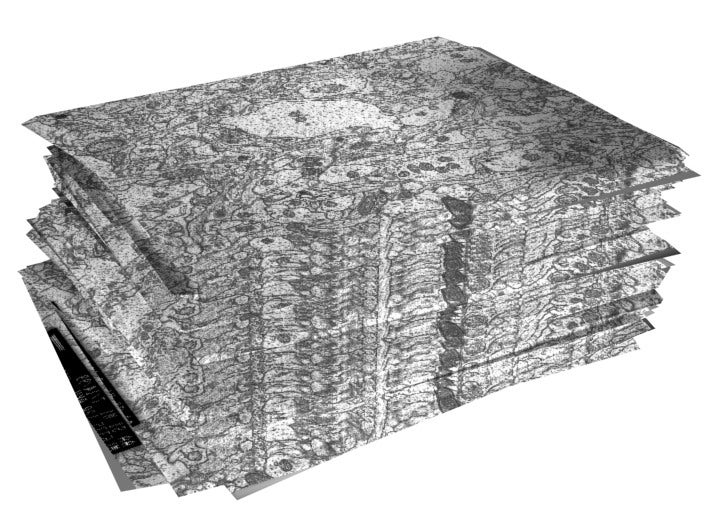
A realigned stack of serial section electron micrographs reconstructs a volume of neuropil from the hippocampus.
Serial Section Electron Microscopy and Three-Dimensional Reconstruction
To overcome the limitations of the inherent two-dimensional nature of electron microscopy, sections can be cut serially from a tissue block. Each section contains a portion of the original structure that can be separately imaged with transmission electron microscopy. The problem then becomes how to reconstruct the three-dimensional structure from these section images.
In the pre-computer era, tracings of sectioned objects were transferred to physical material and assembled, or graphically drawn by hand. Now mostly computer-assisted reconstructions are produced, like the ones in this website, which were produced with the aid of the software developed in our laboratory (see Software).
References:
- Spacek J (1971) Three-dimensional reconstructions of astroglia and oligodendroglia cells. Z Zellforsch Mikrosk Anat. 112:430-442.
- Spacek J, Lieberman AR (1974) Three-dimensional reconstruction in electron microscopy of the central nervous system. Sbor.ved. praci Hradec Kralove 17:203-222.
- Spacek J, Hartmann M (1983) Three-dimensional analysis of dendritic spines. I. Quantitative observations related to dendritic spine and synaptic morphology in cerebral and cerebellar cortices. Anat. Embryol. 167:289-310.
- Harris KM, Stevens JK (1988) Study of dendritic spines by serial electron microscopy and three-dimensional reconstructions. In: Intrinsic determinants of neuronal form and function. Neurology and Neurobiology Volume 37 (Lasek RJ, Black MM eds), pp 179-199. New York: Alan R. Liss. (PDF)
- Harris KM (1994) Serial electron microscopy as an alternative or complement to confocal microscopy for the study of synapses and dendritic spines in the central nervous system. In: Three-dimensional confocal microscopy: volume investigation of biological specimens (Stevens JK, Mills LR, Trogadis JE eds), pp 421-445. New York: Academic Press, Inc. (PDF)
- Fiala JC, Harris KM (2001) Extending unbiased stereology of brain ultrastructure to three-dimensional volumes. Journal of the American Medical Informatics Association. 8(1):1-16. (PDF)
- Fiala JC, Harris KM (2002) Computer-based alignment and reconstruction of serial sections. Microscopy and Analysis. USA Edition. 52:5-7. (PDF)
- Fiala JC (2002) Three-dimensional structure of synapses in the brain and on the web. Proceedings of the 2002 International Joint Conference on Neural Networks, May 12-17, Honolulu, Hawaii, pp. 1-4.